National Instruments discusses Five Technology Trends that Accelerate your Productivity
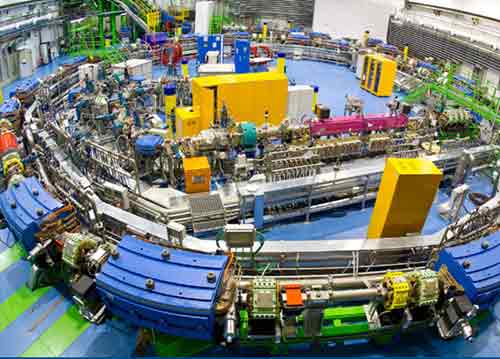
“Since the founding of National Instruments in 1976, our mission has been to equip engineers and scientists with tools that accelerate productivity, innovation, and discovery.
To do this, we closely monitor trends across industries and draw on this insight to develop tools that integrate the ever-increasing power of available technology.
Because NI tools are used in so many different industries and applications, NI is in a position to examine the latest trends in measurement, sensors, networks, test, and more—as they happen. We compiled what we learned in this report to help you take advantage of the latest technological breakthroughs and stay ahead of the competition.
NI isn’t just a supplier—we’re a technology adviser. We hope this information helps you be more productive and focused on what you were hired to do.”
Trends to Watch in 2014
- The Cyber-Physical Design Challenge
- Big Analog Data™—The Biggest Big Data
- The SDRification of RF Instrumentation
- The Evolution of System-Level Design
- Using Mobile Devices as Remote UIs
The Cyber-Physical Design Challenge
Modern engineered systems are rarely designed once, rarely designed in isolation, and rarely ever complete. The braking mechanism in your car evolved from the mechanical lever brake on horse carriages and was soon enhanced with hydraulics to improve braking power and stability. Electrical components were introduced with the advent of power-assisted brakes. Antilock brakes began as mechanical feedback control systems to prevent airplane wheels from locking and eventually migrated to automobiles.
[Cyber-physical systems] can change the way individuals and organisations interact with and control the physical world, and be as revolutionary as the internet. – Dr. Manfred Broy, Founder and Dean of Computer Science, Technical University of Munich
Big AnalogDataTM—The Biggest
In test, measurement, and control applications, engineers and scientists can collect vast amounts of data in short periods of time. When the National Science Foundation’s Large Synoptic Survey Telescope comes online in 2016, it should acquire more than 140 terabytes of information per week.
The SDRification of RF Instrumentation
The modern RF instrument has evolved from merely a measurement device into a premier tool for system design. This evolution was fueled by a broad range of technologies from the software defined radio (SDR). The flexibility of the SDR is revolutionizing not only the wireless industry but also RF test equipment.
Change the Way You Think: The Evolution of System-Level Design
Simply put, a model of computation is one of many languages or development approaches for solving complex issues. Whether it’s exploring new sources of renewable energy or advanced cancer research, the complexity of the problems that today’s engineers face continues to escalate. As a result, a single solution can sometimes require the integration of several models of computation. However, in most cases, engineers are typically trained professional developers in only a single language. To efficiently and effectively solve these increasingly complex issues, the mental model used by today’s engineer must evolve.
Using Mobile Devices as Remote UIs in Measurement and Control Systems
The mobile computing era is in full swing. People now use smartphones and tablets on a daily basis for a variety of useful tasks. Tablet unit sales will soon surpass PC unit sales, something many commentators thought to be a fantasy when Apple announced the iPad in early 2010. Forgetting that these technologies became available only recently is all too easy given how quickly we have integrated them into our lives.
Making Waves in Technology Education
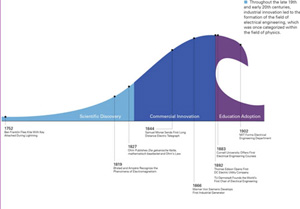
The days of being responsible for a single engineering or scientific domain are ending. The days of designing complete, integrated systems are upon us. Look at the consumer products making an impact on everyday life. Today’s automobiles include more than 70 embedded controllers to tune an engine’s performance on the fly. Some of these automobiles can place and receive phone calls, provide navigation, and drive and park on their own. Basic cellphones are a thing of the past—smartphones are in everyone’s pocket, have millions of applications, and wirelessly connect to a growing array of devices. Using smartphone apps, you can even control home security and lighting while away on vacation. These examples are only the tip of the iceberg.
So what can you learn from today’s innovations to help shape science, technology, engineering, and math (STEM) education and prepare the engineers of tomorrow to solve grand challenges?
First, consider the components of today’s technology innovations to understand what is driving them:
Processing Power Is Abundant—With modern technology like multicore processors and FPGAs, systems can process signals and perform arithmetic in nanoseconds. And history has proven that processors become cheaper and faster every year, allowing for their use in more products than ever before.
Sensors Are Smarter—Taking a physical phenomenon and converting it to an electrical signal has opened doors for millions of applications. Sensors make it possible for electronic systems to hear, see, touch, and act, resulting in automation for countless decisions.
The Software Is the Instrument—Hardware is not merely the physical device anymore. Software drives the functionality of hardware and can transform it into any device you can imagine. The smartphone is a technological feat, considering all the power packed into the palm-sized device, but we all know that the apps are what make it truly revolutionary.
The World Is Connected Wirelessly—Access to the Internet is now ubiquitous in most developed regions and has the power to connect people and devices regardless of their location. The cumbersome wires that have long been the bane of electrical engineers have recently disappeared due to advancements in wireless communication protocols and lower power requirements.
Though all of these components are revolutionary on their own, bringing them together has sparked even greater innovation. Combining the elements of communication, computation, and control into a single system has resulted in the rise of cyber-physical systems. These cyber-physical systems feature many of today’s innovations and require new skills from graduating engineers who are being asked to begin innovating as soon as they enter the workforce.
EVOLUTION THROUGH INNOVATION
Throughout history, changing educational curricula has required the forward-thinking insight of a person, or a small group of people, to identify a need in our society. These pioneers then researched and tested their hypotheses to validate them. If their theories held true, their techniques and strategies were shared with others who replicated them on a greater scale. But these theories and concepts weren’t shared in a chat room or on a message board.
Faculty members in colleges and universities integrated these innovations into their classrooms. These innovations in industry and research inspired the skills required for students to graduate and enter the workforce as the engineers and scientists society needed to build bridges that did not collapse and airplanes that could travel across the Atlantic Ocean on a single tank of fuel.
An example of this can be found in the genesis of electrical engineering as a dedicated field of study, separated from the study of physics, in the 19th century. But this was not just a singular occurrence. In recent decades the trend has repeated itself as biomedical engineering became an official field of study to fill the industry demand of needing engineers with cross-disciplinary knowledge of electrical and mechanical engineering with an understanding of anatomy and medical practices. Other fields such as green engineering, environmental engineering, and power electronics have grown in popularity over the past decade as the world has shifted away from fossil fuel dependency and looked for other ways to harvest and distribute power.
As current students progress through their educations and prepare for industry, cross-disciplinary and design courses have become increasingly important. Ohm’s law and the laws of thermodynamics are not going to disappear, but learning to work in teams and assemble larger, more complex systems comprised of cyber and physical elements is proving to be of greater importance. Students must be able to meet the exciting challenges of applying theory and “doing engineering” to produce results within a single semester, much like they will need to do in industry upon graduation
NEW LESSONS PRODUCE NEW RESULTS
The tools students use in their studies must enable their education in the fundamentals of engineering and science, so they can combine these fundamentals into more complex systems and be relevant to industry to avoid any redundant or wasted knowledge. Some colleges and universities around the world have already begun adapting their curricula to prepare their students to be the cyber-physical system designers that industry now demands. Mechanical engineering students at the University of Leeds in the United Kingdom start with basic control algorithms and then finish with fully autonomous vehicles. The University of California, San Diego has begun a master‘s program that incorporates hands-on model-based design of wireless, embedded, and cyber-physical systems. And students at Olin College recently designed a fully autonomous sailboat using the latest commercial technology with plans to sail across the Atlantic Ocean.
Though focusing curricula on system design requires adjustments to classroom tools and educational goals, professors have found that many incoming students handle these adjustments because they began practicing system design concepts at an early age. In-school robotics clubs and programs like FIRST (For Inspiration and Recognition of Science and Technology) and the WRO (World Robot Olympiad) expose students to the fundamentals of system design in primary school and secondary school. These young engineers construct robots, acquire data from sensors, automate decisions, and control actuators. This is encouraging because these students are preparing to be the well-rounded, multidisciplined, cyber-physical system designers of tomorrow. Given the proper tools, projects, and guidance, they will continue to build these skills as they progress through their educations.