The worldwide focus on energy conservation in the last decade has led to tremendous growth in smart meter deployments, which help manage energy distribution and consumption much more efficiently than traditional utility meters. The smart meter is a central device that bridges in-home energy management systems to long-range back haul communication to utility companies. Utility companies increasingly require frequent two-way communication so they can provide pricing information to consumers based on their specific demand rather than a fixed “peak/off-peak” tariff that is more common today. This smart metering technology enables consumers to make better-informed decisions on when to use major appliances in their homes.
The choice of communications technology for a smart meter is a major decision that requires careful consideration of various design choices. The main factors in choosing the optimal communications technology are cost, security, regulatory compliance, range and power consumption. Several communications technologies are available for smart meter connectivity such as Wi-Fi, Bluetooth, ZigBee, power-line communication (PLC) and sub-GHz wireless. Each technology has its own benefits and disadvantages depending on the application. For long-range applications such as the backhaul communication from the meter to the utility, sub-GHz technology is the most popular choice because of its wireless range, and for this reason sub-GHz deployments are increasing each year.
The United Kingdom, France and Italy are leading the way in smart metering with deployments of gas meters in Europe that are expected to be in the range of 40 million units by 2018. While a portion of these deployments in the UK will use ZigBee in the 2.4GHz band, the majority of these smart metering systems will use sub-GHz wireless communications in the169MHz and868MHzfrequency bands.
According to a recent report by Pike Research, the installed base of smart meters in China will grow from 139 million units in 2012 to 377 million units by 2020. The deployment will be split between various wired and wireless technologies, and a large portion of these meters will be enabled by sub-GHz wireless devices such as Silicon Labs’ Si4438 EZRadioPRO transceiver, which covers the 470-510MHz AMR band in China.
Wireless Benefits for Smart Metering
The obvious advantage of wireless connectivity for smart metering is that there is no need for expensive cabling and associated wireline infrastructure. RF connectivity also makes it easier to retrofit traditional meters with wireless communications modules.
Another key benefit of wireless connectivity is remote “drive-by” meter reading capabilities, which helps the utility reduce labor costs. Instead of individually reading each meter at its physical location, utility employees can simply drive through neighborhoods and wirelessly gather meter data. Error detection and correction in wireless metering systems are also easier and less expensive than with wired systems. Figure 1 shows a typical wireless smart meter system connected the premises to the utility.
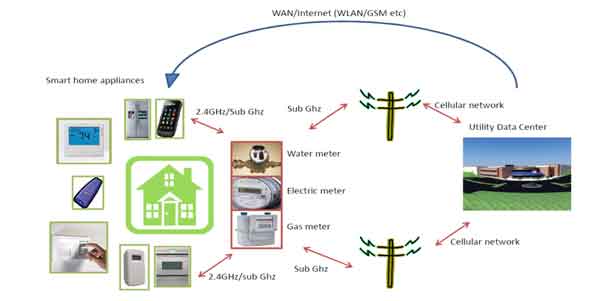
Let’s take a closer look at some of the key considerations in designing a sub-GHz wireless solution for smart metering.
Sub-GHz Wireless vs. Other Wireless Solutions
When it comes to wireless communications, smart meter designers have several choices. Wi-Fi and Bluetooth are ubiquitous and available in several flavors such as 802.11a/b/g/n/ac. The Bluetooth standard is continuously evolving to support low-energy modes with the emergence of Bluetooth Smart Energy. Wi-Fi is ideal for high-bandwidth applications, and Bluetooth is well-suited for very short range (~10m) applications. However, Wi-Fi and Bluetooth are not a good fit for the relatively low-bandwidth and long-range requirements of smart meter applications. ZigBee is a popular choice in the 2.4 GHz band for home automation and security, as well as for mesh networking applications that require a robust wireless link that supports lower power and low data rates. ZigBee also complements sub-GHz in smart metering systems in that ZigBee-enabled devices in the home, such as smart thermostats, can communicate seamless with the smart meter, which in turn communicates with the utility’s metering aggregator through a longer-range sub-GHz RF link.
Wireless Range
One of the primary advantages of using sub-GHz wireless in any application is the long-range capability of this frequency band compared to 2.4GHz or 5GHz. Long-range systems reduce the cost of deployments as fewer concentrators or data collectors are required to serve the same number of smart meters. RF waves at lower frequencies can travel longer distances for a given output power and receiver sensitivity. This phenomenon can be seen by using the Friis formula for path loss and is governed by the laws of physics. As a general rule of thumb, a 6dB increase in link budget will double the range in an outdoor, line-of-sight environment.
As wireless system manufacturers try to squeeze every last dB of performance to get the best link budget, it is important to take into consideration other parameters to make an informed decision on trade-offs. In the commonly used GFSK modulation, lower data rates offer better sensitivity and hence longer range. However, the time to transmit a packet at lower rates means that the transmitter and receiver will have to stay in active modes for a longer period of time, which can increase the overall power consumption. Increasing the transmit power of the radio is an easy way to increase range (“whoever shouts the loudest wins”),but this approach comes at the cost of higher power consumption. While several wireless IC vendors provide solutions with an integrated power amplifier (PA), the efficiency of the PA is a key differentiator. For example, Silicon Labs’ Si446x EZRadio PRO transceivers output 10dBm while consuming 18mA or 20dBm for 85mA.
As range tests are highly sensitive to the environment and device parameters, it is often tricky to achieve an accurate, apples-to-apples comparison between different vendors’ RF transceiver solutions. Care should be taken to ensure that the radio parameters such as frequency, transmit power, bandwidth, packet structure, antenna and method of calculating Packet Error Rate (PER) are all comparable. Forward Error Correction (FEC) and spread spectrum methods such as Direct Sequence Spread Spectrum (DSSS) can help improve the robustness of the RF link under certain conditions, but they come at the cost of increased design cost and complexity due to additional processing requirements.
Table 1 shows ideal link budget and transmission times for different data rates based on currently available transceiver solutions.
Table 1.Wireless Link Budget and Transmission Speeds
Data Rate |
Link Budget with +20dBm Tx |
Time to Transmit 1500 bytes |
500kbps | 117dB | 0.024s |
100kbps | 126dB | 0.12s |
9.6kbps | 136dB | 1.25s |
500bps | 146dB | 24s |
Ultra-Low Power
Another major design consideration for a wireless smart meter is power consumption. While power consumption is acritical concern for battery-powered water and gas meters, it is becoming an important factor for electric meters as well. Battery-powered meters typically use Lithium-Thionyl-Chloride (LiSoCl2) batteries, which need to last from 15-20 years with low duty cycle operation. These LiSoCl2 batteries are significantly more expensive than the cost of the other components in the smart meter (roughly 7X-10X the cost of the transceiver IC). Material and labor costs involved in replacing spent batteries frequently are also much higher than the cost of adding expensive batteries upfront.
In smart meter systems, embedded components tend to spend a majority of time in low-power sleep or standby states so the current consumed in this state needs to be extremely low in the range of tens of nA. The active transmit and receive currents also need to be low especially at low data rates as they lead to longer transmission and reception times. PA efficiency is a key parameter that affects link and power budgets. A higher transmit power will increase the communication range at the expense of battery life. In addition, some other parameters that should be considered during wireless system design are the state transition times to wake up and go back to sleep and the ability of the radio to autonomously duty cycle the device without interrupting the host microcontroller (MCU) for every wakeup and sleep event.
Another factor affecting system power consumption is the ability of the radio transceiver to offload the host MCU from typical packet handling functions such as preamble and sync word detection, Manchester coding and CRC calculations. Performing a majority of these functions in the radio allows the host MCU to spend less time processing the packet and frees up memory and MIPS to perform other functions or remain in a low-power state.
Several MCUs feature an integrated DC-DC converter, which can help increase battery life of the system. Care should be taken in power management and board design that the DC-DC converter does not degrade receiver sensitivity due to switching noise.
Silicon Labs’ EZRadio PRO transceivers, for example, consume only 50nA in standby mode and 10mA in active receive state. As communication from the meter is not continuous and happens periodically, the low standby and receive currents are a key benefit. In addition, a fully configurable low duty cycle mode and packet handler is supported in the device, enabling extremely low system power consumption. Figure 2 shows the chip-level architecture of a typical wireless transceiver that can be used in wireless smart metering designs.
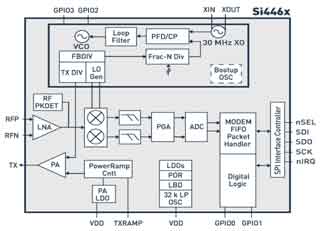
Standards Compliance
One of the more challenging aspects of using sub-GHz wireless technology is regulatory and standards compliance. For a designer trying to create a worldwide smart meter product, the 2.4GHz band is available globally, and only the transmission power needs to be adjusted based on the region. However, sub-GHz frequencies vary depending on the region, making it more challenging for hardware and software designers. The ISM band, which is typically where sub-GHz radios operate, is license free. Each country allocates spectrum independently, and some of the common smart meter frequencies are shown in Table 2 and Figure 3.
Table 2. ISM Frequency Bands by World Region
Region | Main Frequency | Regulatory Body and Standard |
USA | 902-928MHz | FCC part 15.247 |
Europe | 169 MHz and 868MHz | ETSI EN 300-220 / Wireless M-Bus |
Japan | 920MHz | ARIB T-108 |
China | 470-510MHz | SRRC |
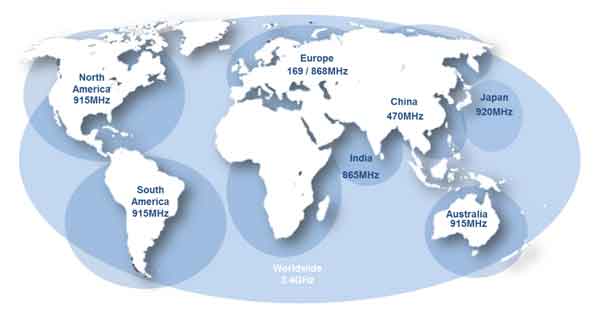
Licensed sub-GHz bands are available in several countries for utilities that may be concerned with interference from other wireless devices. Typically these licensed bands have more stringent regulatory compliance requirements.
For example, in the United States, FCC part 90 applies to a licensed frequency band around 460MHz. Compliance with certain spectrum mask requirements such as mask D requires extremely good phase noise and narrowband performance at low data rates. A number of sub-GHz transceivers, such as Silicon Labs’ Si446x devices, meet the regulatory requirements in this frequency band as well.
The typical approach for most smart meter designs is to create a dedicated hardware board for each region, which simplifies the front end matching network with a cost-optimized board. At a high level, the software protocol is common for each manufacturer, but the low-level PHY parameters must be optimized for each region to meet regulatory standards requirements.
Significant care must be taken to design a fully compliant solution with the lowest possible BOM cost. Harmonics, spurs and transmitter power levels across voltage and temperature have caused many a sleepless night for designers. To ensure success at the compliance testing lab, it’s critical to provide accurate power control and filtering on the board to minimize emissions.
Packet lengths, data rates and protocol choices such as frequency hopping and number of channels are largely constrained by regulatory requirements.
In addition to regulatory compliance, designers must be aware of several wireless standards and industry alliances in various stages of development such as IEEE 802.15.4g/e, Wireless M-Bus, ZigBee and Wi-SUN. The industry is moving towards a standards-based wireless solution based on IEEE 802.15.4 (g/e), which will eventually lead to more choices for consumers as it will enable interoperability among end products. This interoperability ultimately will give consumers the freedom to choose their preferred smart meter products, as well as the utility that provides their energy. In Europe, for example, the Wireless M-Bus specification is the only available standard for wireless meter communication. In the rest of the world, proprietary implementations dominate the deployments today. A challenge for suppliers is the lack of an independent body that certifies devices in the sub-GHz RF market. Today, the onus is on wireless IC suppliers to guarantee wireless device operation and standards compliance based on their own internal testing.
Conclusion
The ease of use and lower costs of standards-based, sub-GHz wireless systems provide compelling benefits in favor of sub-GHz radio usage in smart metering applications. While Europe and the US have led the way in implementing sub-GHz technology in metering systems, the high-volume growth in this market is yet to come from emerging economies such as the BRIC nations. China and India, the world’s two most populous countries with a huge need for energy-efficient solutions, are experiencing a growing trend toward the adoption of sub-GHz wireless communications in meters.
As we see an increase in deployment of sub-GHz wireless smart meters, an ecosystem of home automation and other connected personal devices will develop around these metering systems,using a combination of various wireless protocols. As today’s smart grid merges with tomorrow’s Internet of Things, wireless smart meters will play a key role in delivering cost-effective, secure and easy-to-manage energy to consumers whilegiving them the ability to monitor and control their energy consumption through ubiquitous smart phones and tablets.
About the Author
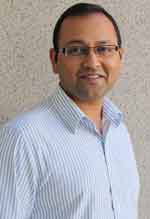
Vivek Mohan, Senior Product Manager, Wireless Embedded Systems, Silicon Labs
Vivek Mohan is a Senior Product Manager for wireless connectivity products including sub-GHz radios in Silicon Labs’ Embedded Systems group. He joined Silicon Labs in 2010 and previously served as an Applications Engineering Manager for short-range wireless products focused on Silicon Labs’ EZRadio and EZRadioPRO transceiver product families. Prior to Silicon Labs, he held applications engineering and design verification roles for wireless SoC products at Marvell Semiconductor. Mr. Mohan holds a Master of Science in electrical engineering from the University of Southern California.