Trends That Could Keep Semiconductor Innovation Going
Simran Khokha, Semiconductor Expert Professional
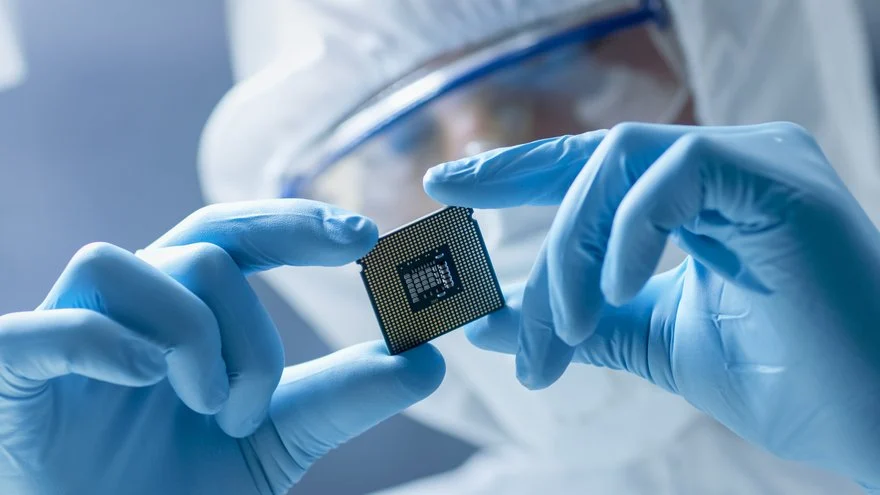
Moore’s Law has been a cornerstone principle guiding the semiconductor industry since its articulation in 1965 by Gordon Moore, co-founder of Intel. This empirical law posited that the number of transistors on a microchip would double approximately every two years, a prediction that has driven exponential growth in computing power and efficiency for decades. However, as the industry approaches the physical limitations of silicon—the material primarily used in chip fabrication—the rate of advancement prescribed by Moore’s Law is slowing. Transistor sizes are nearing the atomic scale, where quantum effects and other physical phenomena challenge further miniaturization. This deceleration is prompting the industry to explore alternative approaches to maintain the pace of semiconductor innovation, reshaping the technological landscape of processors and integrated circuits.
Understanding Moore’s Law and Its Limitations
Moore’s Law has been a prediction and a driving force behind the semiconductor industry’s research and development strategies. It has underpinned the dramatic increases in processing power that have fueled the rise of modern computing and digital technologies. The principle implies that with the doubling of transistor density, computers can potentially become more powerful, smaller, and less expensive to produce at a predictable pace.
However, as transistors shrink to just a few nanometers wide, they encounter significant physical and economic hurdles. One major challenge is quantum tunneling, where electrons pass through physical barriers within the transistor at subatomic scales, leading to leakage currents and power inefficiencies. This phenomenon disrupts the transistor’s ability to function correctly as a switch, impacting the chip’s overall performance and reliability.
Economic challenges are equally daunting. The cost of building fabs capable of producing more minor and complex chips escalates with each generational advance, requiring billions of dollars in investment. The sophistication of technology needed to design and manufacture chips at these scales also pushes the limits of current photolithography and material science, leading to increased production costs and reduced yields.
Emerging Technologies Beyond Moore’s Law
As the semiconductor industry grapples with the diminishing returns of Moore’s Law, several emerging technologies offer promising avenues for continued innovation. These technologies, including quantum computing, 3D stacking of chips, and the use of advanced materials like graphene, propose solutions to the limitations of traditional silicon-based transistors.
Quantum Computing: Quantum computers leverage the principles of quantum mechanics to process information in fundamentally different ways from classical computers. Instead of bits, quantum computers use quantum bits or qubits, which can exist simultaneously in multiple states. This ability allows them to handle exponentially larger datasets and perform computations at speeds unachievable by traditional computers. Quantum computing could bypass some of the physical limitations of miniaturization by focusing on increasing computational power through state superposition and entanglement rather than merely increasing the number of transistors.
3D Stacking of Chips: Another innovative approach is 3D stacking, a technology that layers semiconductor chips vertically rather than expanding them horizontally. This method effectively multiplies the area available for transistors within the same footprint, enhancing data transfer rates and reducing power consumption. 3D stacking can alleviate the scaling limitations by packing more components in a smaller space without the need for further miniaturization.
Advanced Materials: Graphene and other two-dimensional materials represent a significant breakthrough in material science. Graphene, with its remarkable electrical, thermal, and mechanical properties, has the potential to outperform silicon in transistor fabrication. It is stronger, more flexible, and conducts electricity more efficiently than silicon, potentially leading to faster, thinner, and more flexible electronics. Other materials like molybdenum disulfide (MoS2) are also being researched for their semiconducting properties, which might be better suited to ultra-small scale transistors.
Role of Software and System-Level Optimizations
As semiconductor scaling approaches physical and economic barriers, the industry increasingly focuses on software improvements and system-level design optimizations to enhance computational performance. These strategies are crucial in maximizing the capabilities of existing hardware and will play a pivotal role as we transition beyond Moore’s Law.
Software Optimizations: Software plays a critical role in dictating how hardware resources are utilized. Optimizing software involves refining the code and algorithms to run more efficiently on existing processors, thus squeezing out better performance without changing the underlying hardware. For instance, optimizing compilers and adopting more efficient coding practices can significantly reduce applications’ computational load and power consumption.
System-Level Design Optimizations: It involve rethinking the entire system architecture to improve performance and efficiency. It includes the integration of specialized processing units like GPUs and FPGAs (Field-Programmable Gate Arrays) which can handle particular tasks more efficiently than general-purpose CPUs. This approach, known as heterogeneous computing, leverages the strengths of different types of processors to handle diverse workloads more effectively, thereby reducing bottlenecks and enhancing overall system performance.
Integration of AI and Machine Learning: AI and machine learning are increasingly being integrated into the semiconductor design process to manage complex computations and optimize hardware deployment. Machine learning algorithms can predict and manage system loads, dynamically adjusting power use and task allocation to optimize performance and energy consumption. Furthermore, AI can help design chips themselves, predicting outcomes based on different design choices, thereby reducing the time and cost associated with R&D.
By advancing software techniques and system-level designs, the semiconductor industry can continue to drive performance improvements in line with consumer and business demands. These optimizations compensate for the slowing pace of hardware advancements and open new avenues for innovation in computational technologies. As we look beyond Moore’s Law, the synergy between hardware capabilities and software intelligence will be crucial in shaping the future of computing.
Future Outlook and Industry Implications
Looking beyond Moore’s Law, the semiconductor industry is poised for transformative changes driven by emerging technologies and new methodologies. Over the next decade, these developments will likely significantly reshape the economic, societal, and technological landscapes.
Economic Impacts: Transitioning beyond Moore’s Law opens new economic opportunities and challenges. The adoption of advanced materials and innovative manufacturing techniques may initially increase costs but could lead to substantial gains in performance and energy efficiency, offering a competitive edge to early adopters. Additionally, the shift could stimulate job creation in emerging tech sectors while requiring a re-skilling of the current workforce to meet the demands of new semiconductor technologies.
Societal Impacts: The societal implications of advancing semiconductor technology are vast. Enhanced computing capabilities will further enable the proliferation of smart devices and IoT, impacting everything from healthcare to transportation. The democratization of high-performance computing could lead to more personalized services and an increase in the quality of life through technology. However, these advancements could also exacerbate digital divides if access to the latest technologies remains uneven across different regions and socioeconomic groups.
Technological Impacts: Technologically, moving beyond Moore’s Law promises to break the current limits of processing power and energy efficiency. This could accelerate the development of artificial intelligence, virtual reality, and other data-intensive applications by providing the necessary computing infrastructure to efficiently handle complex calculations and massive data sets.
Conclusion
The journey beyond Moore’s Law is about sustaining the pace of semiconductor innovation and redefining the boundaries of what these technologies can achieve. The potential for quantum computing, 3D chip stacking, and using materials like graphene illustrate a future prosperous with possibilities. However, realizing these possibilities will require ongoing innovation and close collaboration within the semiconductor industry. Stakeholders must continue to push the envelope of scientific research and technological development to keep pace with the expanding demands of the digital world. This collective effort will ensure that the semiconductor industry remains at the forefront of global technological advancement.
About Author
Simran Khokha has half a decade worth of experience in upcoming Consumers Product tech in major semiconductor Giants, including several years in product development and designing within APAC region (Singapore) and now Product management and Marketing in Europe region (Germany), focusing across a host of segments including Automotive, IoT, and Consumer Electronics. She has previously worked in Design of first of it’s kind System On chips used for AI/ML, self driving cars and future applications. Herexperience expands over companies like AMD, STMicroelectronics and Infineon.