Ever-tightening automotive fuel emission standards are becoming challenging. The gasoline engine needs the help of an electric motor to meet these standards, leading to the introduction of mild hybrid electric vehicles (MHEV) with higher battery voltages. 48V hybrids are in production today and are proliferating. A48V buck converter with integrated MOSFETs in an advanced CMOS process helps meet these standards by with standing high-voltage load-dump transients and operating with low EMI, low duty cycles, and high efficiency.
Your car may be on its last leg and you are in the market for a new one. Are you considering another gasoline engine car, a full electric vehicle (EV), or something in between? With so many flavors of hybrid available, such as full hybrid electric vehicle (FHEV), plug-in hybrid electric vehicle (PHEV), or mild hybrid electric vehicle (MHEV), there are many options to choose from. Feels a bit like ordering your favorite flavor of latte, doesn’t it?
The trajectory of the automobile engine begins with the gasoline engine and ends with an electric motor. The force behind this dramatic transition is the necessity to meet ever stricter fuel emission regulations. Currently, the gasoline engine uses the aid of an electric motor to help with both start-stop and regenerative braking applications, but its use will eventually fade. We project that automobiles will be fully electric 20 years from now.
A total ban of gasoline vehicles is expected around 2040. Strong candidates to accompany this transformation are the 48V mild hybrid electric vehicles (MHEV), which help older gasoline engines meet emission standards at a modest cost (Figure 1).
In this design solution, we will review the architecture of an MHEV and propose a buck converter that not only works with 48V batteries while withstanding high input-voltage load-dump transients but operates with low EMI, low duty cycles, and high efficiency.
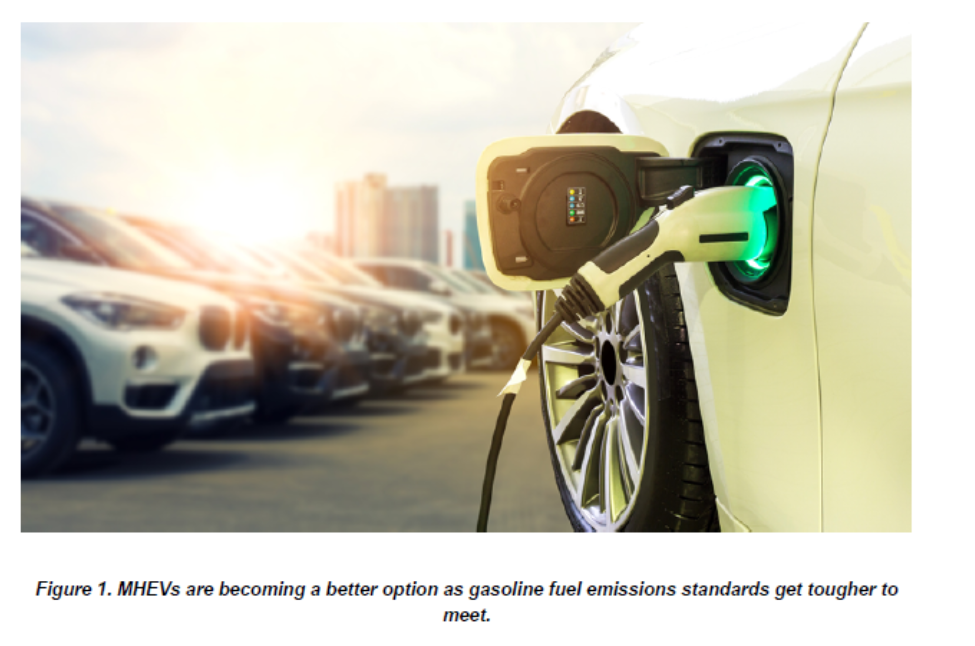
48V Auxiliary System
The MHEV belt starter generator (BSG) provides greater efficiency by replacing the alternator and starter motor of traditional cars with a single device which assists the powertrain (Figure 2).
During braking, the energy flows from the combustion engine to the 48V battery. The engine applies torque to the BSG, which in response, acts as a generator. The electric waveforms generated by the BSG are rectified by the three-phase inverter via the IGBT or MOSFET intrinsic diodes, producing the DC current that charges the 48V battery.
During start-stop, the energy flows from the 48V battery to the BSG, which acts as a motor. In this phase, the BSG draws power from the 48V battery through the three-phase inverter power transistors. A DC-DC converter bucks the 48V down to 16V to power the 3-phase inverter gate drivers, providing the proper motion sequence to the BSG.
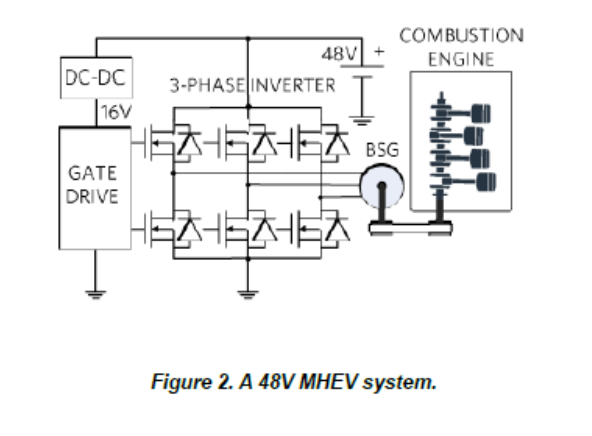
The BSG starts the engine during start-stop, provides torque boost to improve acceleration performance, and charges the battery during braking. The 48V battery can also power accessories like fans, pumps, electric power steering racks, compressors, and aid with start-stop systems. A 48V battery can deliver the same power of a 12V battery with ¼ of the current, reducing the car electric wiring losses, size, and weight.
48V Battery
Let’s take as an example, a 1kWh, 48V, 21Ah lithium-ion MHEV battery. According to the “VDA 320 – Electric and Electronic Components in Motor Vehicles 48V On-Board Power Supply” recommendation, the battery has an unlimited voltage operating range between 36V and 52V and allows limited operating modes between20V and 60V and dynamic overvoltage up to 70V. The maximum operating voltage (60V) is the maximum permissible contact voltage safe for human operators so that the system is not classified as “high voltage” with the risk of electrical shock.
48V Buck Converter Robustness
As discussed above, the 48V buck converter can be subject to voltage spikes as high as 70V and for as long as 40ms, a very long time for electric stress. Operation at or above this limit can permanently damage the device. Accordingly, the buck converter input voltage’s absolute maximum rating must have enough margins above 70V for safe operation.
Low EMI
The ECU’s power management electronics must withstand harsh automotive environments and be protected from electromagnetic interference (EMI). Accordingly, the 48V buck converter must meet the CISPR25 Class5 specifications for EMI. Fixed-frequency converters help with the spikes during both radiated and conducted emissions testing. Fixed and adjustable frequency allows design engineers to focus the filtering on a certain frequency to help pass EMI tests. By contrast, constant on-time architectures typically exhibit variable frequency making it much more difficult to have good EMI performance.
Front-End 48V Buck Converter
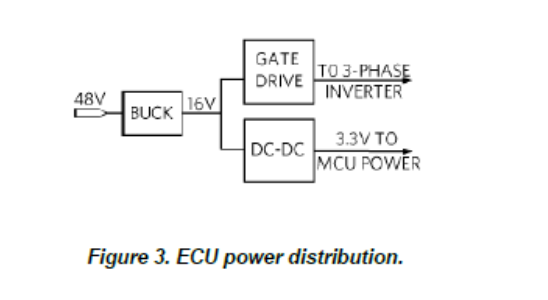
The automobile has dozens of electronic control units (ECUs). The typical ECU’s power management architecture is shown in Figure 3. The 48V interfaces a robust front-end buck converter that withstands the battery’s static and dynamic voltage conditions and in turn helps power various gate drives for motor control with a 16 to 20VOUT, while also providing back-up power to MCUs in case the 12V battery gets disconnected.
Compared to 12V input, the 48V buck converter will tend to have higher switching losses, a problem that can be mitigated by reducing the frequency of operation (f) and adopting an advanced process with smaller minimum features and lower parasitic capacitances (C). In addition, the control technique must lend itself well to operation with low duty cycles. For example, a 16V output and a 48V input lead to a . This means that the buck converter high-side transistor conducts only 33% of the time while the low-side transistor conducts 67% of the time. Such considerations can guide the sizing of the power transistors for optimum performance.
Integrated Solution
MAX20059 is a high-efficiency, high-voltage, synchronous step-down DC-DC converter IC with integrated MOSFETs that operates over the 4.5V to 72V input voltage range. With an 80V absolute maximum rating, the IC has a margin of 8V vs. 72V operating condition, and a 10V vs. 70V battery dynamic overvoltage recommendation.
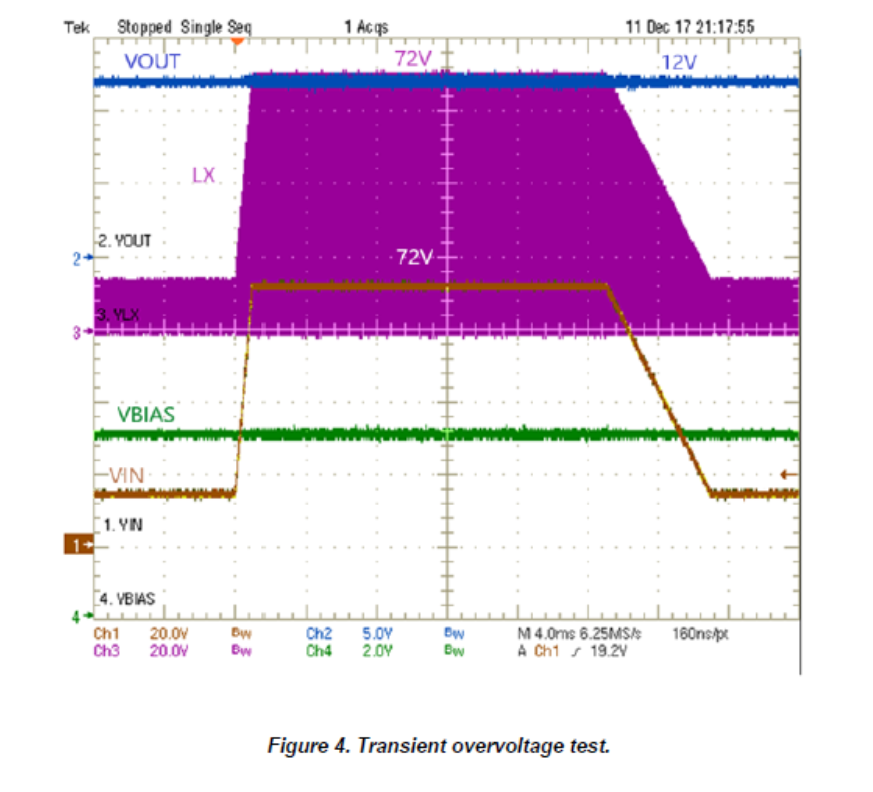
The IC is thoroughly tested according to VDA320 recommendations. As an example, Figure 4 shows one of the tests, the transient overvoltage test, under conditions exceeding VDA320 recommendations:
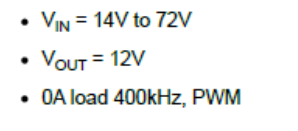
The IC is also tested for electromagnetic radiation according to EMI CISPR25 Class 5 specifications. As an example, Figure 5 shows one of many EMI tests, performed with a 200MHz to 1GHz log-periodic (horizontal)antenna. The IC level of emissions are well below the limit.
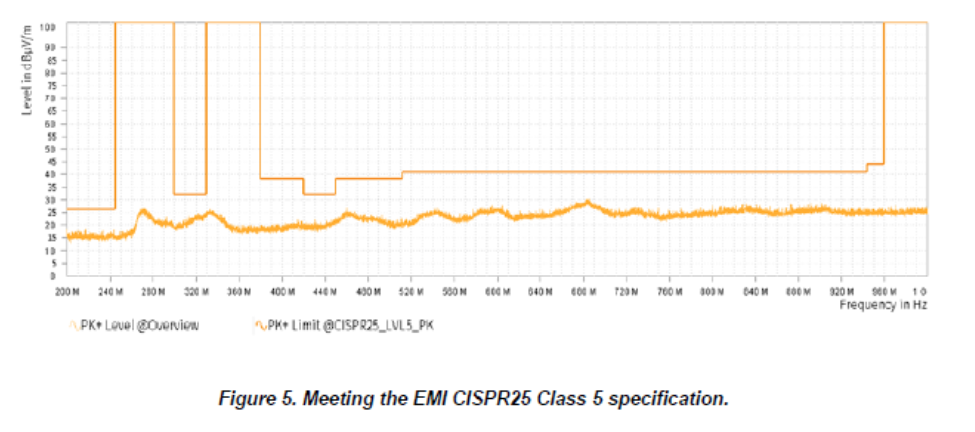
The converter delivers up to 1A current. Output voltage is programmable from 0.8V to 90%VIN. The feedback voltage-regulation accuracy over -40°C to +125°C is ±1.5%. The IC features a peak current-mode control architecture and can be operated in the pulse-width modulation (PWM) or pulse-frequency modulation (PFM) control schemes. The IC is available in a 12-pin (3mm x 3mm) side-wettable TDFN package with an exposed pad for thermal heat dissipation. Figure 6 shows the 48V to 16V typical application circuit.
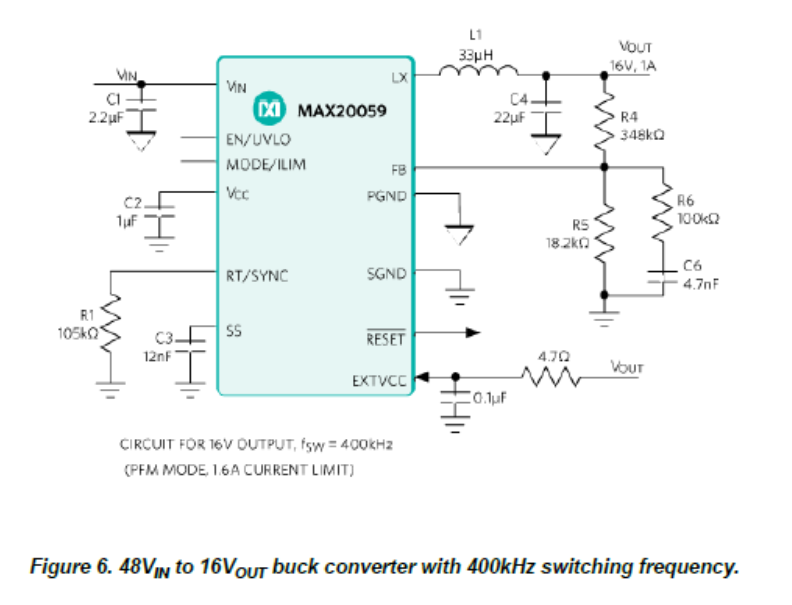
High Efficiency
For maximum flexibility the IC has two modes of operation. PFM provides high efficiency across the operating range, but the output-voltage ripple is higher compared to PWM. PWM operation (Figure 7) provides constant frequency operation at all loads and is useful in applications sensitive to switching frequency. Accordingly, the switching losses weigh higher at light loads and the MAX20059 can achieve > 90% peak efficiency from48V to 16VOUT.
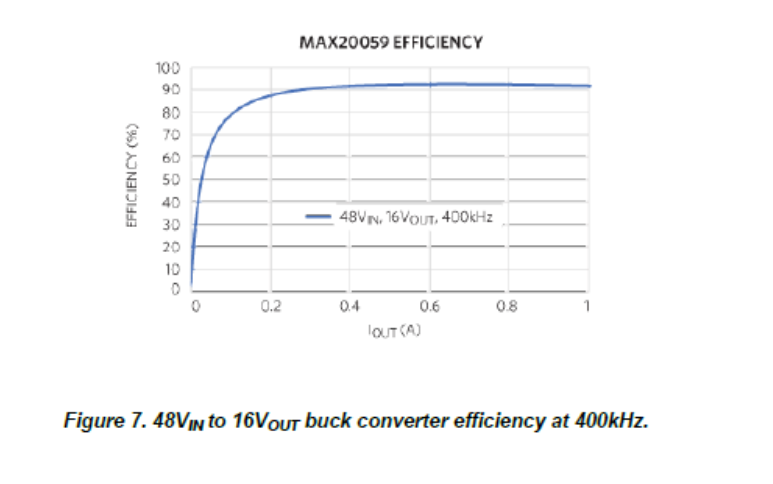
Conclusion
The automotive environment is undergoing an epic transition. Due to the ever-increasing severity of fuel emission standards the gasoline engine is on a descending parabola that will eventually end with a fully electric car. In the meantime, hybrid implementations, like the 48V MHEV, are proliferating. We highlighted the challenges of designing voltage regulators that operate within the harsh 48V automotive environment and presented a high-voltage front-end buck converter that has high tolerance to input voltage static and dynamic variations, low EMI, and high efficiency.
A similar version of this design solution originally appeared on Electronic Products on February 05, 2020.