Instruments that simulate the irradiance and spectral output of our own Sun in order to test photovoltaic solar cells in indoor environments.
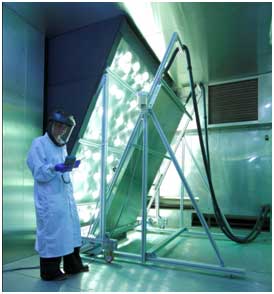
A solar simulator allows us to test solar systems independent from current weather conditions and time of day. In other words, solar research can happen at an accelerated rate because of the amount of time able to study a system with constant testing conditions. The simulator creates artificial sunlight using xenon-arc lamps. These lamps produce similar quality light to that of the sun. With the help of parabolic reflectors, this light is made into direct beam radiation with intensity close to 1 sun. By making direct beams of light we are able to test systems that concentrate sunlight as well as those that do not. The lights are arranged in an array to produce a large area for testing. Several types of lamps have been used as the light sources within solar simulators. This area is adjustable by adding or subtracting lights in the array but is ultimately limited by the size of the room. The IEC 60904-9 Edition2 and ASTM E927-10 standards are a common specification for solar simulators used for photovoltaic testing. The light from a solar simulator is controlled in three dimensions, Spectral content, spatial uniformity and temporal stability.
Classification of Solar Simulators
A solar simulator makes possible the accurate measurement of the optical-to-electrical conversion efficiency of solar photovoltaic (PV) cells. The instrument quantitatively simulates the irradiance and spectral output of our own Sun so that PV manufacturers can obtain efficiency data without leaving panels to bake in unpredictable outdoor environments. For performance criteria of solar cells and modules (such as conversion efficiency, quantum efficiency, and current-voltage characteristics) to be quantitatively consistent across hundreds of manufacturers, standards for solar simulators have been developed. The primary standards organization that details solar simulator performance requirements, specifications, and test protocols is the International Electro-technical Commission. The specific standards—IEC 60904-9 ed2.0, ASTM E927-10, and JIS C 8912—classify solar simulators with a three-digit “Class XYZ” designation, where X defines the spectral match (the ratio of the actual percentage of total irradiance to the required percentage specified for each wavelength range), Y defines the irradiance spatial non-uniformity, and Z defines the irradiance temporal instability for the solar simulator. That is, a Class ABB solar simulator meets Class A spectral match, Class B spatial non–uniformity, and Class B temporal instability specifications.
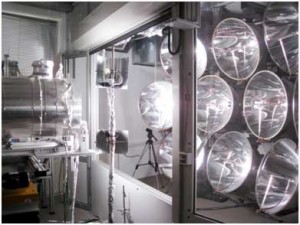
For spectral matching, each standard specifies the percentage of total irradiance from the solar simulator that must fall within each 100 nm wavelength interval between 300 and 1100 nm in order to best mimic the spectral output of the Sun. This wavelength range was historically selected to cover the typical absorption region for silicon considering that most early PV cells were single-crystal silicon; however, it does not address the performance of concentrating photovoltaic (CPV) systems that use III-V semiconductor materials with absorption out to 1800 nm and some exotic thin-film PV products. Not all solar simulators are created equal. The class differences in solar simulators are largely due to differences in the system optics and the simulator light source.
Solar simulators installed globally
High-tech indoor solar simulator in University of Minnesota
In a windowless solar energy lab in the Mechanical Engineering Building at the University of Minnesota, researchers are tapping the power of a new piece of high-tech equipment that can replicate the amount of sunlight equivalent to more than 3,000 suns. A concentrating solar simulator, the first of its kind in the nation, was used by researchers in the university’s College of Science and Engineering in a variety of experiments, including converting concentrated sunlight, carbon dioxide and water into synthetic hydrocarbon fuels. The indoor lab will provide consistent experimental conditions independent of weather and time of day. Based on concepts of similar simulators in Europe, University of Minnesota researchers designed the $450,000 piece of equipment to provide concentrated light equivalent to that possible from modern solar optical systems. The investment is already paying off. The lead researchers at the university have secured nearly $1 million in research money from a new National Science Foundation Emerging Frontiers in Research and Innovation grant in collaboration with CalTech and UCLA. The University of Minnesota’s Solar Energy Laboratory researchers also have received nearly $1.4 million in grants from the Initiative for Renewable Energy and the Environment within the university’s Institute on the Environment.
Solar Simulator facility at Centre for Sustainable Technologies of the University of Ulster
The Centre for Sustainable Technologies (CST) recently commissioned a large scale state-of-the art solar simulator. This unique indoors solar simulator facility including climate control features allows performance evaluation and/or product certification of:
- Photovoltaic (PV) Modules
- Solar Thermal Collectors
- Solar Concentrators
- Advanced Evacuated Glazing
- Energy Storage
- Advanced Insulation Materials
The simulator has been designed to simulate sunlight of similar intensity and spectrum. It provides uniform light on a test area of dimensions up to 2m by 1.5m with a target distance between 1.8m and 2.2m. The simulator lamp array consists of high power 35 metal halide lamps arranged in 7 rows of 5 lamps each. Each lamp is equipped with a rotation symmetrical paraboloidal reflector to provide a light beam of high collimation. In order to achieve uniform distribution of light intensity on the test area, a lens is inserted in each lamp to widen the illumination of light. The combination of reflector-characteristics, lens and lamps ensures a realistic simulation of the beam path, spectrum and uniformity. The unique design feature of the simulator is to achieve uniform intensity of light on vertical, horizontal as well as inclined surface. This feature allows full testing of solar concentrating systems to be carried out as every direction of the sunlight incident on the concentrator aperture can be simulated. The simulator is equipped with an external control unit. The unit includes safety features to protect the system from power failure and overheating. Any fault in the system will be displayed on the simulator control panel. The intensity of the light on the test target can be controlled automatically or manually through the control unit. A suitable frame structure has been designed to provide support and full mobility for the lamp frame. The frame enables tilt adjustment of the lamp array to any angle from horizontal to vertical and automated height adjustment from ground level to six meters above ground. The frame is also able to move along the laboratory floor allowing testing of all test arrangements in it. A secondary frame has been constructed to house the “cool sky” double filter glass system required to remove infrared and UV parts from the simulated light.
Solar Simulator in ETH-Zentrum, Zurich
The High-Flux Solar Simulator provides a rapid external source of intense thermal radiation that approaches the heat transfer conditions of highly concentrating solar systems. The light source is a high-pressure argon arc enclosed in a clear quartz envelope. It delivers up to 100 kW continuous radioactive power with a peak flux intensity exceeding 5000 kW/m2(equivalent to a solar concentration of 5000 suns). The arc envelope is internally cooled by using a spiraling film of de-ionized water that rapidly flows between the plasma arc and the clear quartz lamp tube. The arc produces radiation at visible wavelengths with additional power in the infrared and ultraviolet regions of the spectrum. Advanced optical filters can be applied to the tubular quartz envelope for adjusting the spectral distribution of the arc to closely match the suns true output – either to AM0 (space) or AM1.5 (terrestrial) standards. The system was manufactured by Vortek, Canada. This light source is closed-coupled to precision optical reflectors of elliptical shape to produce an intense beam of concentrated radiant energy. With this arrangement it is possible to achieve power flux intensity equivalent to 5000 suns (1 sun = 1 kW/m2) and process temperatures exceeding 3000 K. Power flux intensities and temperatures can be adjusted to meet the specific requirements of the application by simply varying the position of the test target along the axis of the focusing mirrors or by varying the electrical input power to the arc electrodes.
Solar Simulator in IIT Bombay – ECE Department
The Solar Thermal Simulator is developed by Indian Institute of Technology Bombay (IITB) as a part of the project titled “Development of a Megawatt-scale Solar Thermal Power Testing, Simulation and Research Facility”, sponsored by the Ministry of New and Renewable Energy (MNRE), Government of India. Essentially, the solar thermal simulator solves energy and mass balance equations for user defined plant configurations. The main features of the simulator are graphical user interface for data input and output, manual as well as database entry of climatic and equipment parameters, overall plant optimization through multiple simulations, user defined time step and time horizon for the simulation, etc. The simulator produces results, such as, output of all equipments for each time step, annual power generation, capital cost and cost of energy. The results can be displayed in tabular form and/or graph form as well as the same can be exported to MS Excel file. Parametric study can be done by changing the system parameters, such as, control variable, location, stream parameters and equipment model parameters.
The main features of the simulator include graphical user interface for data input and output, simulation of user defined Process Flow Diagram (PFD), library of climatic and equipment parameters or facility for manual entries, provision for overall optimization through multiple simulations, user defined time step and time horizon for the simulation, etc. The interface of the simulator is self-describing hence very user friendly and easy to use. The results can be displayed in tabular form or graph form and also can be exported as MS Excel file. The different equipment used in solar thermal power plant such as solar collector, heat exchanger, pump, storage vessel, turbine, auxiliary boiler, etc., are incorporated into the simulator library.
LED – Based Simulator from Erlangen, Germany
Researchers at the Bavarian Center of Applied Energy Research (ZAE Bayern) and the University of Erlangen-Nurembergare exploring an LED-based solar simulator with improved spectral matching capabilities.3Unfortunately, while most lamp-based simulators achieve Class A spectral match, deviation from the solar spectrum is still significant—especially in the 800–1000 nm window where crystalline silicon has its peak spectral response. The prototype LED simulator uses 700 specially designed ultrabright LEDs in 22 different wavelengths from 400–1100 nm. Preliminary results show that it successfully simulates the Am1.5 spectrums with a Class A spectral match, can be operated in both steady state or pulsed mode, and offers lower power consumption and longer lifetime than its lamp-based counterparts. Look out, legacy simulator manufacturers: The era of LED-based solar simulators may be on the horizon.