Designing and simulation of military radars has to be done not only considering the articles the radar were meant to detect but also all kinds of affecting factors. Today’s military radars are designed to be smart enough to differentiate a coming bird with a bird structured drone to similar app wise. In this exclusive article by EM Media we have bought to you some technologies from across the world discussing latest tech in Designing and Simulating Military Radars.
Military radar is required to detect all kind of articles in its range precisely. Depending on the characteristics of the surveillance area and targets, deployment of different types of long and short range radars systems became must. Plus to surveillance, military radar must detect unknown target in the surveillance area, covering the whole area under the detection range of the system. To achieve military security targets it is required to deploy costly sensors all over the area old school style. This could work with UAVs in public areas by high tech in military and defense needs advanced solutions. Here’s comes in picture the “modeling and simulation” of military radars. Today’s military teams are required to operate in environments that are increasingly complex. Such settings are characterized by the presence of ill-structured problems, uncertain dynamics, shifting and ill-defined or competing goals, action/feedback loops, time constraints, high stakes, multiple players and roles, and organizational goals and norms. Warfare scenarios are real world systems that typically exhibit such characteristics and are classified as Complex Adaptive Systems. To remain effective in such demanding environments, defence teams must undergo training that targets a range of knowledge, skills and abilities. Thus oftentimes, as the complexity of the transfer domain increases, so, too, should the complexity of the training intervention. The design and development of such complex, large scale training simulator systems demands a formal architecture and development of a military simulation framework that is often based upon the needs, goals of training. In order to design and develop intelligent military training systems of this scale and fidelity to match the real world operations, and be considered as a worthwhile alternative for replacement of field exercises, appropriate Computational Intelligence (CI) paradigms are the only means of development. A common strategy for tackling this goal is incorporating CI techniques into the larger training initiatives and designing intelligent military training systems and war games. Some of the important CI techniques that are used to design these war-game components are explained with suitable examples, followed by their applications to two specific cases of Joint Warfare Simulation System and an Integrated Air Defence Simulation System for air-land battles is explained. From flight experiments, the uncertainty in aspect angle to a threat sensor, due to aircraft dynamics, is quantified for various aircraft. In addition, the RCS fluctuation behavior of a military jet trainer is investigated by dynamic in-flight measurement. The monostatic and bistatic RCS of an F-117 are modeled and findings show that spline interpolation provides superior accuracy when interpolating the RCS data. Smooth and conservative RCS models are suggested and a new RCS sampling scheme is presented. A model based on experimental data is suggested for determining the range of aspect angles that an aircraft is likely to orient towards a threat sensor, and experimental RCS data is compared to the classical Swerling radar target models. Possible consequences for military operations and the design of military systems are discussed and considerations for modeling the interaction between air defenses and aircraft penetrating those defenses are given.
Gallium nitride (GaN) transmitters, radio frequency (RF) tiles, and solid-state modular 3-D radar are among the latest innovations in next-generation military radar systems to embrace commercial-off-the-shelf (COTS) components to reduce costs. Designers of these systems have also adopted modular design to streamline installation and maintenance.
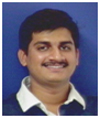
In words of Mr. Pawan Varanasi Manager – Product Support and Application for major RF products (Spectrum Analyzer, Vector Network Analyzers & Signal Generators) Rohde& Schwarz, Today’s radar systems consist of RF electronics, signal processors and appropriate signal processing software. While the development of RF electronics is mainly focused on increased measurement bandwidth and higher data acquisition speed, the main challenge of the signal processing unit is to find improved algorithms being able to take more and more tasks from the RF electronics. This is taken into account with appropriate test instruments which are developed along with internal and external signal processing capabilities. One of the critical parameters for Radar System Performance is Range resolution i.e. the capability to separate (resolve) multiple targets moving in a close distance with same radial velocity to each other. Short Pulses are required for better range resolution and the same can be achieved by pulse compression technique. Pulse compression is a mathematical method to reduce the effective pulse width at constant energy of the transmitted signal at the receiver. A generator provides a signal to the transmit antenna which radiates an appropriate wave to a flying object. The reflected wave Rx, which is delayed and attenuated, sometimes down close to the noise floor of the system, is received, demodulated and then lead to the “correlate”, which compares the transmitted and the received baseband signal. Because we are talking about a coherent system, the transmitter and the receiver are supplied by a common reference frequency.
The R&S vector Signal generator along with R&S® Pulse Sequencer software enables the engineers to perform Radar simulation and modeling in a simple and efficient way. With this Test system it is possible to generate pulsed signals with basic modulation schemes. Signals with simple pulses, pulse trains and repetition of pulses can be generated. In addition, pulse trains with different pulses and pulse breaks can be generated sequentially. All major modulation formats for modulation on pulse are available with internal and external data sources. Typical pulse parameters like rise and fall time, ripple, droop, overshoot, etc., can be defined. Pulse sequences with control elements, antenna diagrams and antenna scans are also available as an enhanced feature on Pulse Sequencer Software. Antenna diagrams and antenna scans can be combined to form an emitter. For scenario simulation, multiple emitters together with a receiver can be placed on a 2D map. This makes it possible to simulate real-world signals as they occur at the RF output of a receiver antenna.
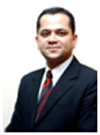
Further Mr. Kaustubh Nande; Country Marketing Head of ANSYS India joined us on this article. He commented radars are integral parts of highly complex systems –be it air-traffic control, air-defense, or ballistic-missile defense systems. The heightened nature of safety and security demanded by these systems make it imperative to evaluate and analyze radar performance, and quantify how radar operations impact system performance. However, using expensive outdoor range testing or hardware simulators to test radar systems is not a cost-effective option. For instance, real aircrafts can cost hundreds of thousands of dollars an hour and the results from the test runs are not repeatable. Also, the interaction between radar and its environment is highly complicated in nature.
Given these factors, simulation is the most rational and practical method of supporting radar system and performance analysis. Radar simulators can simulate ground-based radars or radars on board moving objects such as tanks, ships and aircrafts. Simulations can be set up based on actual data collected, or for hypothetical and/or planned operations. Depending on the sector about 20–25% visionaries and SMEs are investing in simulation technologies. For instance, the automotive industry along with technology giants such as Google is increasingly adopting radar simulation to test next gen self-braking, self-parking, and self-driving cars. Automotive radars must operate in a complex and dynamic environment where interactions with the vehicle and its surroundings can have a significant impact on the performance of the radar system. Typically, radar system performance and interaction among the various components and subsystems are often not discovered until expensive prototypes have been built and testing is conducted in the integration lab. A full system solution that allows engineers to simulate complex 3-D systems and predict system performance and electromagnetic effects using appropriate global and local simulation technology is therefore a critical requirement today. Radar simulation and system performance models can evaluate complex scenarios several hundred times in an hour by using same or different scenarios, while keeping costs down significantly.
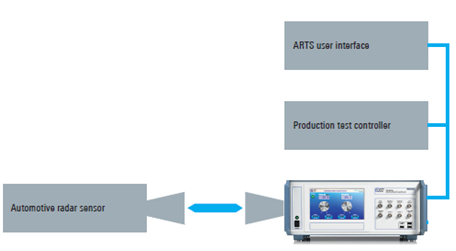
Pavan Varanasi, Rohde & Schwarz shared one of their application studies in relevance to Modeling and Simulation of ground-based, airborne, ship-borne or automotive radar systems. Radar sensors are key components in advanced driver assistance systems (ADAS) and have made possible an entirely new class of in vehicle comfort and safety functions within just a few years. ADAS applications such as brake and lane change assistants and adaptive cruise control take an active role in vehicle guidance and therefore play a major role in safety. The reliability requirements for these systems are correspondingly strict, and thorough field testing is required before they are launched on the market. Lab testing, however, should be conducted prior to field testing in order to anticipate all critical situations using realistic test scenarios. There is an economic reason behind this approach: test drives are costly and time-consuming, while lab tests are relatively cost-efficient, can be quickly executed and repeatable. The ARTS 9510 radar target simulator opens up these advantages for testing in vehicle test system equipped with radar sensors. The ARTS9510 family was developed specifically for the automotive industry, i. e. for radars operating in the 24 GHz and 77 GHz frequency bands. The ARTS meets all current and future requirements for simulated distances, variable target sizes (radar cross section, RCS), resolutions and object speeds. It delivers the same precision for near-range radars such as those used for parking assistants as does for long-range systems, for which it can simulate an object at up to 2.4 km traveling at radial velocities of up to 700 km/h. Optionally it can even display the angle of arrival of moving objects.
Challenges while designing Military Radar System
The main challenge encountered is designing Radar Systems to work in a complex spectral environment with multiple signals from Radar and telecom networks, said, Pavan Varanasi. He further added the validation to design parameters ensures proper functionality of Radar System. An essential part of verifying the overall functionality of the radar is target emulation. Traditionally, radar targets are emulated using a fiber-optic line to introduce a time delay which translates into a target range relative to the radar. An additional I/Q modulator inserts a Doppler frequency shift to emulate a radial target velocity. Although this method is common practice, it lacks flexibility. The time delay cannot be changed easily because the fiber-optic line is of fixed length. It is therefore not possible to emulate time-varying ranges such as occur when the target is moving. Additionally, multiple virtual targets require multiple optical fibers of different lengths, leading to a complex test setup. Rohde& Schwarz offers a novel approach to flexibly and conveniently generate radar echoes. Range and Doppler frequency shift can be controlled and adapted in a straightforward manner to reproduce scenarios with multiple static and moving targets. It generates virtual radar targets by applying time delays, Doppler frequency shifts and attenuations – in real time and reproducibly.
Mr. Kaustubh Nande commented in exclusive talk with EM Media that modern radar systems are extremely complex, comprising complicated architectures and cutting edge technologies from different engineering domains. Testing the performance of such complex systems under a variety of operating conditions is a daunting task as we need test for several potential problems such as interference, jamming, etc. Not only that, we need to ensure rapid simulation and testing to save development time and costs. This means that not only do we have to simulate the radar system; we also need to simulate the entire environment in which it is going to be deployed. In this scenario of increased product complexity, it is crucial for manufacturers to get their offering ‘right the first time’ in order to maintain control over cost, time, and resources. Our platform speeds up the modeling process, enhances integration, performs cross domain analysis and verification, as well as validates systems using real life, complex scenarios to help organizations enhance time to market and reduce costs.
Professional Solutions in Market
Rohde & Schwarz
The radar target generator depicted here consists of an R&S®FSW Signal and Spectrum Analyzer and an R&S®SMW200A Vector Signal Generator. The spectrum analyzer is used for down-conversion and digitalization of the radar transmit signal. It captures the waveform seamlessly and streams the in-phase and quadrature components (I/Q) continuously to the signal generator. The generator is used for signal manipulation and retransmission. An inbuilt fading block in the transmission line, normally used in mobile communication applications, manipulates the I/Q data before up-conversion to the desired radio frequency and retransmission.
ANSYS India
Businesses require comprehensive system solutions that can assemble complex 3D radar systems and predict system performance using appropriate global and local simulation technology. ANSYS offers modern technologies including Integral Equation (IE), hybrid Finite Element with Integral Equations (FEBI), hybrid IE-regions, Physical Optics (Po), and High Performance Computing (HPC). These simulation methods combine the power of advanced computing hardware and sophisticated numerical methods to enable rapid and accurate simulation of complex materials and geometries. We offer an integrated model-based platform for radar simulation and system performance modeling for a wide range of industries including aerospace and defence, and automotive.
Conclusion
With the increasing threats and advances in attack and spying systems, military radars are expected to be just smarter defense tech. This article have discussed and advances in simulation and modeling for modern radar systems and what market is offering.